Using MRI to investigate fluidized bed reactors
- 1. University of Cambridge, Chemical Engineering and Biotechnology, Cambridge, United Kingdom
- 2. University of Canterbury, Chemical and Process Engineering, Christchurch, New Zealand
In this work we show that ultrashort echo time (UTE) MRI can be used to image bubble formation in a fluidized bed reactor. Images of bubble formation were analyzed in conjunction with slice selective velocity measurements to provide new insight into the dynamics of the particulate phase of fluidized bed reactors. There are two key points of interest in this study: first, how bubbles form in fluidized beds, and second, how the rheology of the particulate phase affects bubble formation. A better understanding of these processes will help to improve mathematical models of the systems and thereby improve the energy efficiency of reactors. Though fluidized bed reactors are used in many industrial processes, relatively little is known about their internal dynamics, partly due to the difficulty in studying rapidly evolving, opaque systems. MRI can provide non-invasive measurements of optically opaque systems, however acquiring images of solids with short T2 and T2* relaxation times on the time scale necessary to see bubble formation is challenging.
We have adapted UTE, a technique from medical MRI, to enable snapshot measurements within a fluidized bed [1]. UTE uses a soft, slice selective excitation and samples k-space from the center-out. This makes it possible to minimize the time between the excitation and acquisition, the effective echo time (TE), to that of the system ring down. We are using a TE of 10 μs, which allows for images to be acquired of short signal lifetime, "more solid", materials. In this study we used plant seeds, Nicotiana and Lobelia, as the particulate phase in our fluidized beds. Both seed varieties have approximate relaxation times of T1 = 500 ms, T2 = 25 ms, and T2* = 150 μs. In order to resolve bubbles in our system, our image resolutions were 0.5 mm × 0.5 mm × 2 mm and image acquisition times were on the order of 25 ms. To achieve this spatial and temporal resolution it was necessary to use a tip angle of 10° and to reconstruct the images with a compressed sensing (CS) algorithm. We acquired three types of UTE images in this study: one-dimensional profiles, XY images, and ZY images, where Z is aligned in the vertical direction.
In fluidized beds, bubble size is smallest near the base of the reactor where air enters via a distributor plate. As they ascend bubbles coalesce, resulting in accelerating bubble rise velocities. We measured these velocities using 1D UTE images. This information was then used to predict positions at which to center XY slices, enabling us to acquire multiple 2D UTE image "snapshots" of bubbles as they ascended through the reactor. By acquiring 1D profiles between each XY image we were able to observe bubble coalescence and bubble rise velocities simultaneously.
The "travelling wave" model has been hypothesized as a mechanism of bubble formation, illustrated in Figure 1a [2]. Using UTE MRI in the ZY plane we were able to image this phenomenon, Figure 1b. This is the first time this has been observed experimentally in a 3D fluidized bed.
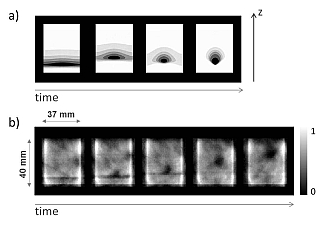
The formation of bubbles predicted by the travelling wave model is sensitive to the granular "rheology" of the sample. The apparent rheology of the granular phase is related to the distribution of the velocity of the particles and the time between collisions [3]. If the time between collisions is long, the apparent viscosity is low, whereas if the time between collisions is short, then the apparent viscosity is high. We are investigating the rheology using a couette cell rheo-NMR device [4] and a spin echo, pulsed field gradient (PFG) NMR experiment, allowing us to measure particle motion over varying observation times. The variance of particle velocity <uz2> is measured from the attenuation at low q. At observation times less than the mean collision time, <uz2> is almost constant with increasing observation time. For observation times longer than the mean collision time the motion is averaged over many collisions, therefore <uz2> decreases. We can estimate the mean collision time from the inflection point at which <uz2> starts to decrease rapidly, as shown in Figure 2. By measuring how the collision time varies spatially we are providing experimental data to validate models of granular flow.
In this study we have developed UTE MRI techniques to rapidly image the solid phase of a dynamic system. Using UTE images and PFG NMR, we have acquired qualitative and quantitative measurements that have improved our understanding of fluidized beds and will inform the design of more efficient and robust systems.
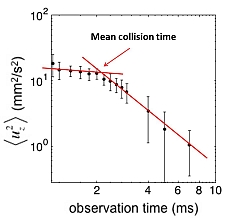
In this work we show that ultrashort echo time (UTE) MRI can be used to image bubble formation in a fluidized bed reactor. Images of bubble formation were analyzed in conjunction with slice selective velocity measurements to provide new insight into the dynamics of the particulate phase of fluidized bed reactors. We have adapted UTE, a technique from medical MRI, to enable snapshot measurements within a fluidized bed [1]. UTE uses a soft, slice selective excitation and samples k-space from the center-out. This makes it possible to minimize the time between the excitation and acquisition, the effective echo time (TE), to that of the system ring down. We are using a TE of 10 μs, which allows for images to be acquired of material with short T2 and T2* relaxation times. Plant seeds with approximate relaxation times of T1=500 ms, T2=25 ms, and T2*=150 μs have been used in this study. In order to resolve bubbles in our system, our image resolutions are 0.5 mm × 0.5 mm × 2 mm and image acquisition times are on the order of 25 ms. To achieve this spatial and temporal resolution it was necessary to use a tip angle of 10° and to reconstruct the images with a compressed sensing (CS) algorithm.
We used 1D images to measure the bubble rise velocity. This information was then used to calculate slice offsets to select an XY slice through the center of a bubble at several locations as it ascended through the reactor. Using this method we were able to image bubble coalescence.
The "travelling wave" model has been hypothesized as a mechanism of bubble formation, as illustrated in Figure 1a [2], and can clearly be seen experimentally in ZY images shown in Figure 1b.
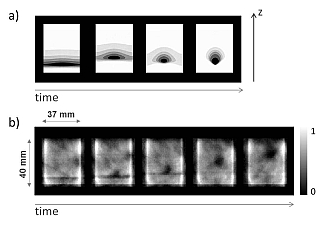
In this study we have developed UTE MRI techniques to rapidly image the solid phase of a dynamic system. Using UTE images and PFG NMR, we have acquired qualitative and quantitative measurements that have improved our understanding of fluidized beds and will inform the design of more efficient and robust systems.
- [1] Fabich, H.T., et al., (2014), Ultrashort echo time (UTE) imaging using gradient pre-equalization and compressed sensing, Journal of Magnetic Resonance
- [2] Glasser, B.J., et al., (1996), One-and two-dimensional travelling wave solutions in gas-fluidized beds, Journal of Fluid Mechanics
- [3] Seymour, J.D., et al., (2000), Pulsed Gradient Spin Echo Nuclear Magnetic Resonance Imaging of Diffusion in Granular Flow, Physical Review Letters
- [4] Brox, T., Galvosas, P., (2015), WO201503063 (A1)